Introduction to actin
Actinis a crucial protein for eukaryotic cells, serving as a fundamental component of the cytoskeleton, providing structural support and shape to the cell. Actin plays a key role in numerous cellular processes, including motility, division, and intracellular transport. With a molecular weight of approximately 43 kDa, actin protein is highly conserved across different species, highlighting its evolutionary importance. There are three major actin isotypes: α-actin, β-actin, and γ-actin. These isotypes share over 90% amino acid homology with each other and over 98% within a given isotype group, with differences occurring mainly in the amino-terminal 30 residues. This region of the protein is located on the periphery of the actin double helix of F-actin and is important for interactions with other proteins, including myosin.
Isotype variation and cell behavior
Actin isotypes behave differently both in vitro and in vivo, reflecting specialized functions within different cellular contexts. For example, γ-actin has been observed to localize differently compared to other isotypes, indicating that the various actin isotypes play unique roles in the cell. This specificity is highlighted by the differential binding of actin-associated proteins to each isotype. A clear example is the protein ezrin, which shows special affinity for β-actin. This highlights the importance of using the appropriate actin isotype in your experimental setting to accurately recapitulate physiological conditions.
Recent studies have demonstrated that isotype-specific interactions of actin-binding proteins (ABPs) can have profound effects on cellular processes. For example, differences in the subcellular localization of γ-actin suggest that certain isotypes are involved in distinct cellular functions, and using the wrong actin isotype may affect the interpretation of experimental results.
Actin polymerization: from G-actin to F-actin
Actin exists in two main forms: globular actin (G-actin) and filamentous actin (F-actin). G-actin is a monomeric, soluble protein, while F-actin is a polymerized filamentous structure.
Polymerization ProcessUnder physiological conditions, G-actin polymerizes by ATP hydrolysis to form F-actin, which is characterized by a double-helical structure that gives the filament its inherent polarity: the plus end (or barbed end) of the filament polymerizes faster compared to the minus end (or pointed end), creating an inherent asymmetry in the filament structure.
Critical Concentration (CC): The actin polymerization process is controlled by the concentration of actin monomers. The critical concentration (CC) is defined as the monomer concentration at which the rates of polymerization and depolymerization are in equilibrium. At concentrations above the CC, actin polymerizes until the free monomer concentration falls to the CC. For muscle actin at 4°C, in the presence of 2 mM Mg²⁺ and 50 mM KCl, the CC is approximately 0.03 mg/ml. In the absence of these ions, the CC increases significantly, showing how ionic conditions affect polymerization. Similarly, non-muscle actins exhibit a range of CC values depending on the ionic environment and temperature, reflecting their dynamic nature.
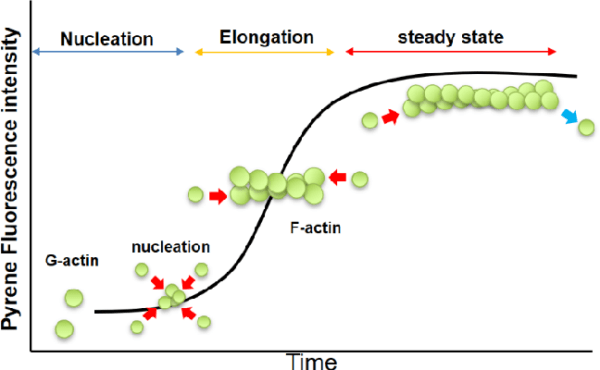
Measurement of actin polymerization
Several methods have been employed to measure actin polymerization, each with specific advantages and limitations.
- Enhanced fluorescence of pyrene conjugatesThis technique uses pyrene-conjugated actin, which becomes increasingly fluorescent upon polymerization. The fluorescence enhancement is up to 20-fold, providing a sensitive and versatile method to monitor actin polymerization in real time. This method is advantageous for studying polymerization dynamics while minimizing sample destruction.
- DNase inhibition assayThis assay takes advantage of the high affinity interaction between G-actin and DNase I, which inhibits DNase activity and allows differentiation of G-actin from F-actin. This assay is particularly useful for quantifying the amount of monomeric and filamentous actin in cell extracts and for studying actin-binding proteins that generate G-actin from actin filaments.
- Viscosity measurements: Viscosity measurements can be performed using high or low shear methods depending on the length of the filament. High shear methods are good for detecting small differences between short and medium length filaments, while low shear methods are used for long filaments. These methods may disturb the filament and may not be as accurate, but they are useful for comparing cross-linking of filaments between samples.
- Spin-down assay: The spin-down assay utilizes differential sedimentation to separate F-actin from G-actin. This method provides quantitative data on actin polymerization at steady state. When used in combination with other methods, it allows quantification of actin polymers over time. However, this method is destructive and may overestimate the amount of actin monomers due to incomplete sedimentation of small actin oligomers.
Engineered actin and its uses
Modified actin, such as fluorescently labeled or biotinylated actin, is a valuable tool in research.
- Fluorescent actinFluorescently labeled actin is used to study actin dynamics both in vivo and in vitro. For example, fluorescent actin can be microinjected into cells to observe actin treadmilling and myosin mobility. This approach is useful for understanding the behavior of actin filaments in different cellular contexts.
- Pyrene actinPyrene-conjugated actin is used to monitor actin polymerization. Upon polymerization, pyrene-actin fluorescence increases, providing insight into the dynamics of actin filament formation.
- Biotin ActinBiotinylated actin has many applications, including investigating actin dynamics and selective purification of actin using streptavidin-coated beads, and can also be used as a probe for actin dynamics within cells when combined with streptavidin- or avidin-coated gold particles.
Actin-binding proteins (ABPs)
Actin interacts with a variety of proteins called actin-binding proteins (ABPs), which greatly influence its function. Over 150 ABPs have been identified, representing approximately 25% of cellular proteins. These proteins regulate actin dynamics and play important roles in various cellular processes.
- Muscle contractionActin filaments interact with myosin to drive muscle contraction, an interaction essential for muscle function and movement.
- Cell motilityActin-based motility is crucial for cell migration, enabling cells to move and change shape in response to various signals.
- CytokinesisDuring cell division, actin filaments form a contractile ring that separates daughter cells, ensuring proper cell division.
- Cytoplasmic streamingThe actin network supports cytoplasmic flow within cells and contributes to a variety of intracellular processes.
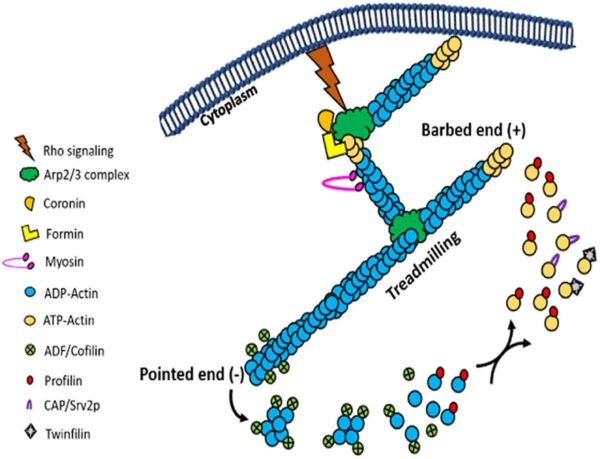
ABPs can be isolated and studied using a variety of biochemical, genetic, and immunological techniques. Methods such as affinity chromatography and pyrene-actin fluorescence-based assays are useful for identifying and characterizing these proteins.
Medical significance of actin research
Actin research has great medical implications, especially in understanding diseases associated with cytoskeletal dysfunction. Key areas of interest include:
- Erythrocyte membrane proteinsMutations in proteins involved in the red blood cell membrane skeleton, such as spectrin, ankyrin and band 3, cause conditions such as hereditary spherocytosis and elliptocytosis. These mutations affect the shape and stability of red blood cells, leading to increased fragility and lysis.
- Muscular dystrophies: Dystrophin, an actin-associated protein, is essential for linking the actin cytoskeleton to the muscle cell membrane. Mutations in dystrophin cause Duchenne and Becker muscular dystrophy, which are characterized by progressive muscle weakness and degeneration. Understanding the role of dystrophin in muscle cells is crucial for developing therapeutic strategies for these diseases.
- Neurodegenerative diseases: Proteins associated with the actin cytoskeleton are also involved in neurodegenerative diseases. For example, proteins that link the actin cytoskeleton to synaptic structures are essential for maintaining neuronal function. Dysregulation of these proteins may contribute to diseases such as Alzheimer’s and other neurodegenerative disorders.
Molecular Basis and Dynamics
Actin forms complex ultrastructures that support cell shape and movement. The cytoskeleton, composed of actin filaments, provides scaffolding for cellular tissue and facilitates a variety of cell movements. Actin filaments are involved in the formation of the pseudopodia that amoebas use to crawl and the microvilli of enterocytes that extend into the digestive tract for nutrient absorption.
The dynamic nature of actin is a hallmark of its function. Actin filaments are continuously assembled and disassembled in response to the changing needs of the cell. This dynamic behavior is controlled by ATP binding and hydrolysis. Free actin monomers bind ATP and associate with growing filaments. When ATP is hydrolyzed to ADP, actin filaments undergo subtle structural changes, become less stable, and ultimately disassemble. This dynamic process, known as treadmilling, involves continuous polymerization at one end and depolymerization at the other end, allowing the filaments to “move” through the cell without changing their overall length.
Controlled growth of actin filaments
Within cells, actin filament growth is tightly regulated to prevent uncontrolled polymerization. Proteins such as gelsolin and profilin play key roles in controlling actin dynamics. Gelsolin severs actin filaments, generating new ends for further polymerization, whereas profilin binds to actin monomers and promotes their incorporation into filaments. This control ensures that actin filament formation responds to cellular signals and needs, maintaining cellular integrity and function.
Future directions of actin research
Advances in actin research are uncovering new aspects of actin function and regulation. Future research directions include the following:
- Structural studiesHigh-resolution structural studies of actin and actin-associated proteins provide deeper insight into their interactions and functions. Techniques such as cryo-electron tomography and X-ray crystallography are instrumental in elucidating these structures.
- In vivo studiesAdvanced imaging techniques, such as live-cell fluorescence microscopy and super-resolution microscopy, are enabling a better understanding of actin dynamics in living cells and tissues.
- Drug DevelopmentTargeting actin dynamics and interactions with specific inhibitors or modulators holds promise for developing therapeutic strategies for diseases associated with cytoskeletal dysfunction.
- Systems BiologyIntegrating data from different omics approaches will enable understanding of the complex network of actin and its interacting partners, providing a comprehensive view of the role of actin in cellular processes.
In summary, actin is a versatile and essential protein involved in a variety of cellular functions. Its dynamic nature and interactions with numerous proteins give actin a central role in cell biology. Ongoing research into its structure, function, and regulation continues to provide valuable insights into both fundamental cellular mechanisms and the development of actin-related diseases.
Integrin signaling: a short review – The Science Notes